MODEL ORGANISMS
Alternatives to the mouse and threadworm
Mice, fruit flies and threadworms populate innumerable research labs across the world. But besides these ‘classical’ lab animals, there are dozens of even more unusual model organisms – some of which are only now becoming popular. Each of them has its own advantages and disadvantages, as our selection here explains.
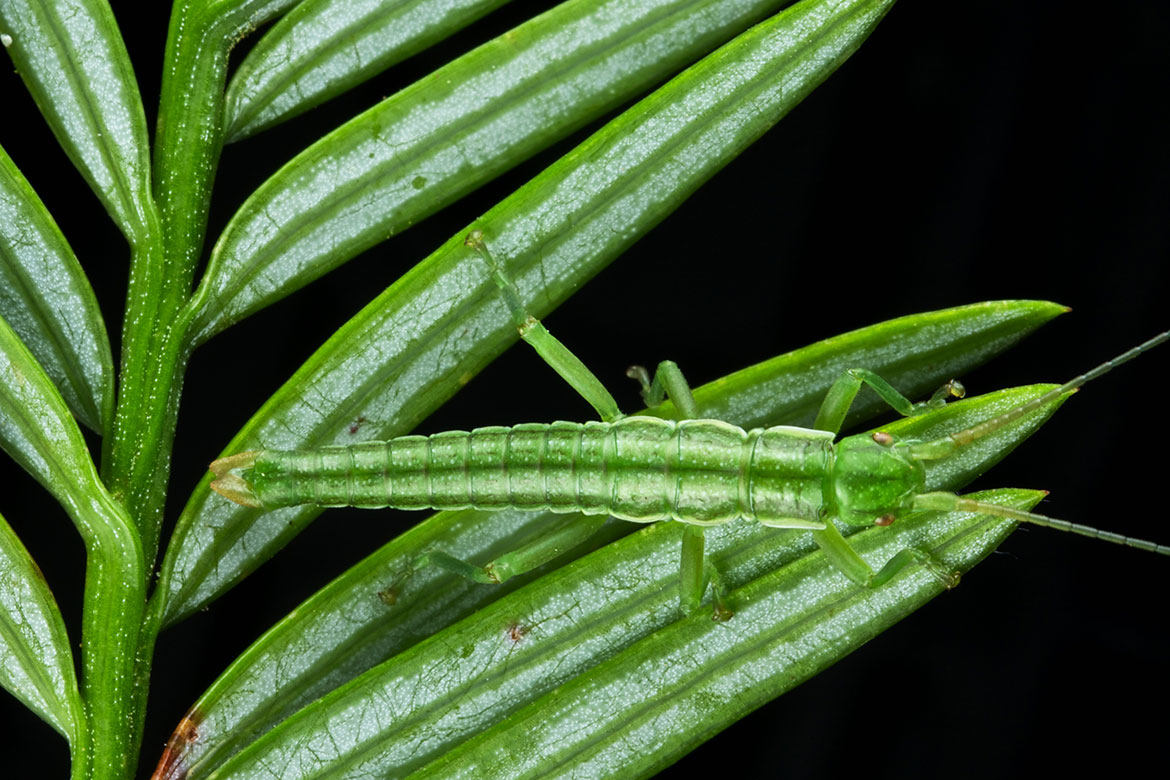
Just two laboratories in the world – one of which is in Switzerland – are researching into the stick insect of the genus Timema. | Photo: zVg
Why do so many animal species take the trouble to engage in sexual reproduction? And what prompts others to forgo males? Stick insects of the genus Timema might be able to answer this question. Tanja Schwander leads a research team of ‘sexperts’ – her own name for them – at the University of Lausanne, and is investigating ten species of this earwig-like insect.
“In the course of its evolution, asexual reproduction has occurred several times among Timemas, independently of each other”, says Schwander. Among its different species, there are always two that are closely related: the one reproduces sexually, the other asexually. Schwander’s studies have shown that the constant reconfiguration of their genetic material by means of sexual reproduction means that a species reproducing this way adjusts quicker to changes in the environment.
The Timemas are an exciting model organism, says Schwander. “But sometimes I do wish I were working with fruit flies”. They reproduce in less than two weeks, and thousands of research groups work with them. Timemas, however, only produce one generation a year, and only two laboratories in the world work intensively with them. So it takes a long time for experiments to conclude, and the researchers working with them have to cope with a general lack of experience, routine and genetic tools.
But Schwander thinks it is important that there should be more model organisms than the five or six most commonly used in labs, “if only because you can’t reflect the whole variety of Nature with just lab mice, fruit flies and yeast”. She is also somewhat sceptical about support programmes for new model organisms, such as the USD 24 million initiative currently being run by the National Science Foundation (NSF) in the USA. “Researchers themselves have to find out what system is best for their projects and research questions, and they have to be motivated to work with it”.
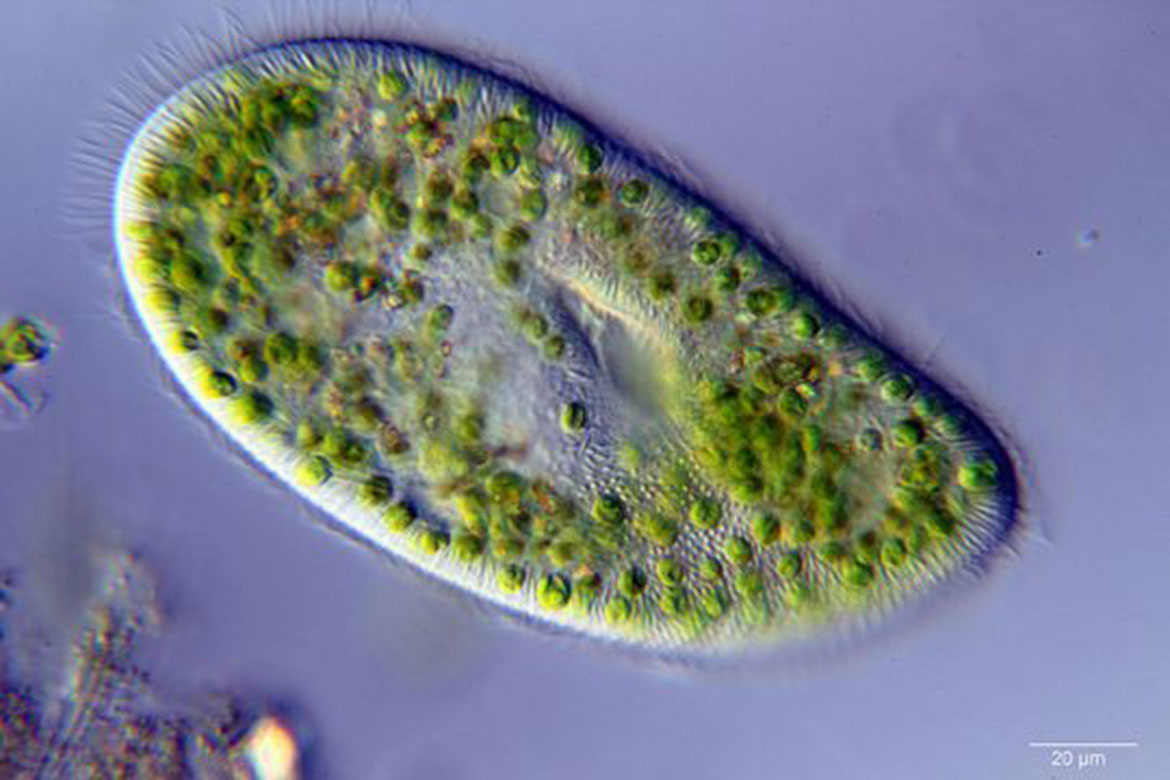
It’s brightly lit here under an optical microscope. But otherwise, the slipper animalcule leads something of a shadowy existence. | Photo: zVg
Cut-and-paste genetic artists
Slipper animalcules are popular objects of study in school biology lessons. They are relatively large, transparent, single-cell organisms whose organelles can be observed through an optical microscope. But as a model organism it leads something of a shadowy existence. This is a shame, says Mariusz Nowacki of the University of Bern. “Its size means you can use it to conduct excellent studies of a lot of cell processes”, he says. Nowacki himself is looking into how slipper animalcules cut up their genetic material and rearrange it when they divide. Similar processes also take place in human cancer cells.
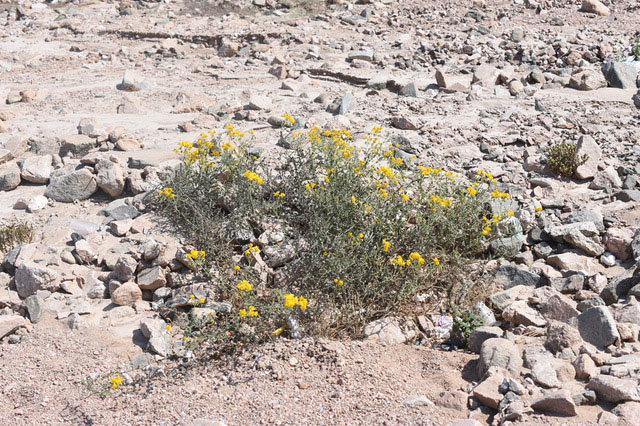
Just a wallflower? No, the wild tomato Solanum offers valuable insights into how species emerge. | Photo: zVg
Creating species in isolation
Today, we know of 13 species of wild tomato, some of which can cross with each other. These include two closely related species whose genetic material is currently being investigated by Simon Aeschbacher of the University of Zurich. Their distribution areas are largely divided from each other by the Peruvian coastal desert, which prevents them from fertilising each other. But in some places, their distribution areas intersect, allowing a genetic exchange to take place. This offers an excellent opportunity to find out how distance and habitats can influence the emergence of species.
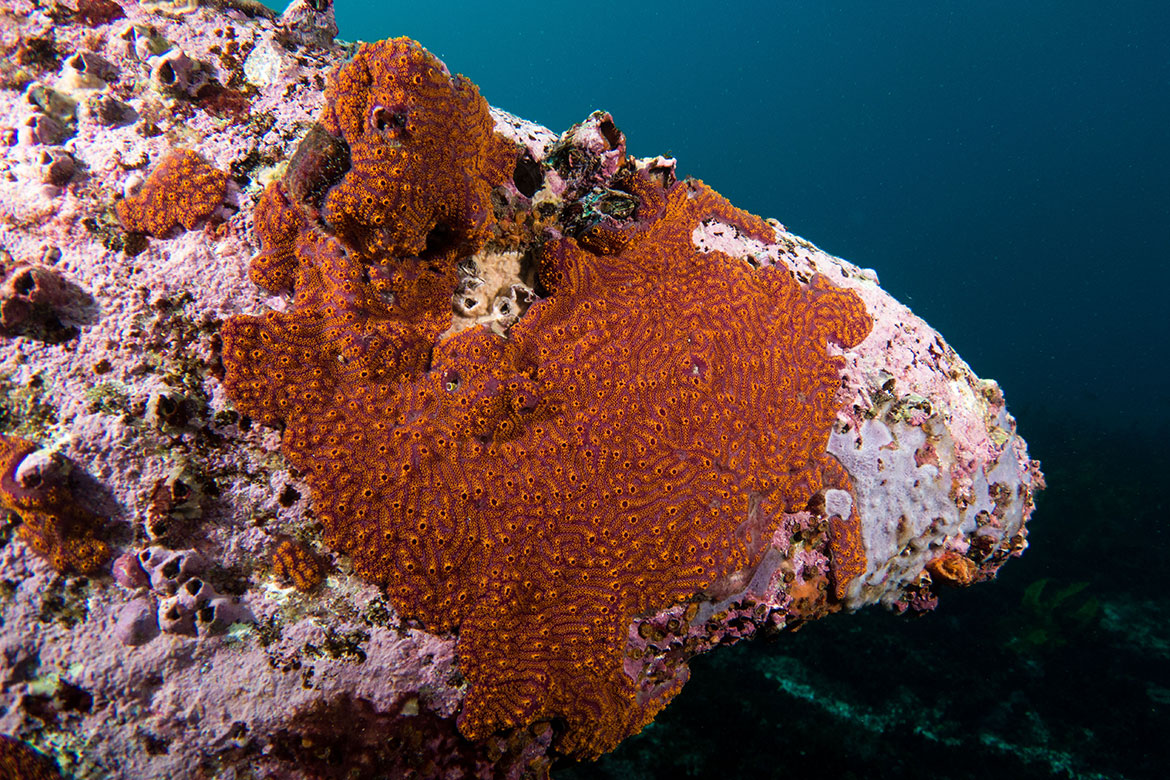
The tunicates of the genus Botrylloides are difficult to keep in the lab. | Photo: John Turnbull
Regenerative secrets of the deep
Tunicates are regarded as the closest relatives of the vertebrates – but they can do something that no vertebrate can do. Even the smallest fragment of their vascular system can regenerate into a whole animal. Simon Blanchoud of the University of Fribourg is investigating this whole-body regeneration in a colony of Tunicates of the genus Botrylloides. The individual animal is barely three millimetres in size. In Blanchoud’s lab, these tiny sea-dwelling creatures live on glass plates. He is delighted with them: “You can use them to investigate a multitude of fundamental biological questions”, he says. But they also pose challenges. For example, you cannot control when they sexually reproduce in the lab. Keeping them also brings its own problems. “All the other labs that work with Botrylloides are near the sea and have access to normal sea water. Our culture is in artificial sea water and offers a more controlled environment – but we don’t know exactly if this bothers the animals over a longer period of time”.
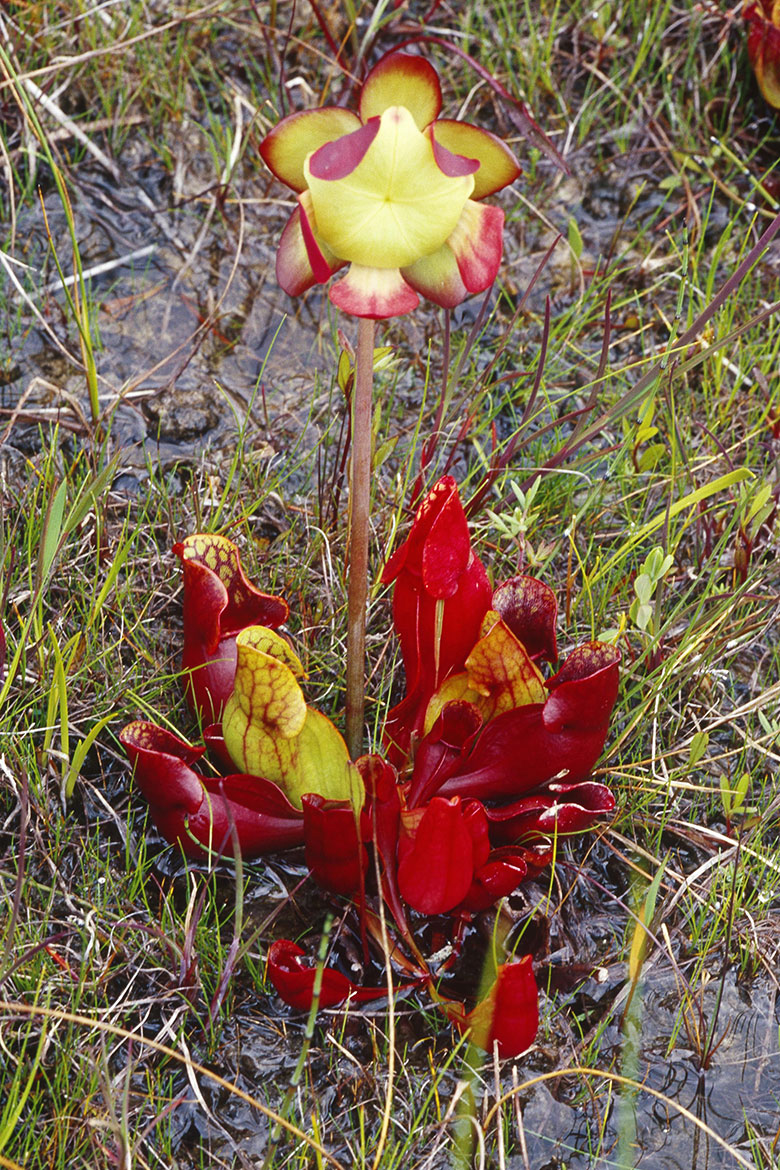
First it was imported to Switzerland, then it found its way into Swiss labs: the purple pitcher plant Sarracenia purpurea. | Photo: Ray Coleman/SPL/Keystone
A microcosm in a pitcher
The leaves of the purple pitcher plant are tubed-shaped and collect rainwater – along with insects that fall into them and drown. Bacteria break down these insects, which then become prey to single-cell organisms called protists. Louis-Félix Bersier of the University of Fribourg is studying the co-occupants of these meat-eating plants from North America.
“It’s a simple model that enables us to study processes in a natural ecosystem”, says Bersier, who used to study ecosystems on meadows around the Lake of Neuchâtel. “In Neuchâtel, we had 12 fields with 144 study areas, hundreds of species and even more interactions between them all – that was time-consuming”. When he switched to his new system, two chance occurrences proved helpful: A student applied to join him who already happened to have experience with pitcher plants. And he also found out that this plant had already been brought to Switzerland. He now carries out experiments on pitcher plant ecosystems out in the field, and brings back samples to examine in his lab.
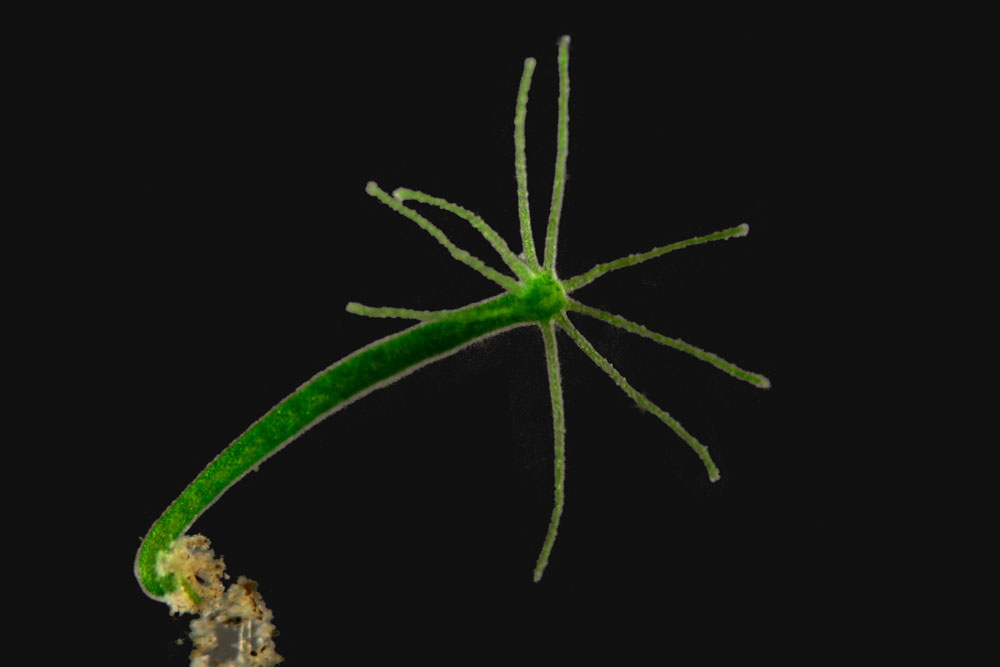
The freshwater polyp Hydra vulgaris is also studied in schools. | Photo: zVg
A pouch full of stem cells
“The Hydra”, says Brigitte Galliot, a researcher at the University of Geneva, “is a pouch full of stem cells”. If you take good care of them, they are almost immortal, and ideal for studying how wounds heal, along with tissue regeneration and ageing. For 30 years now, Galliot has been working with these solitary freshwater polyps – and her enthusiasm for them has not diminished. The hydra is a fascinating animal that can awaken everyone’s curiosity, she says.
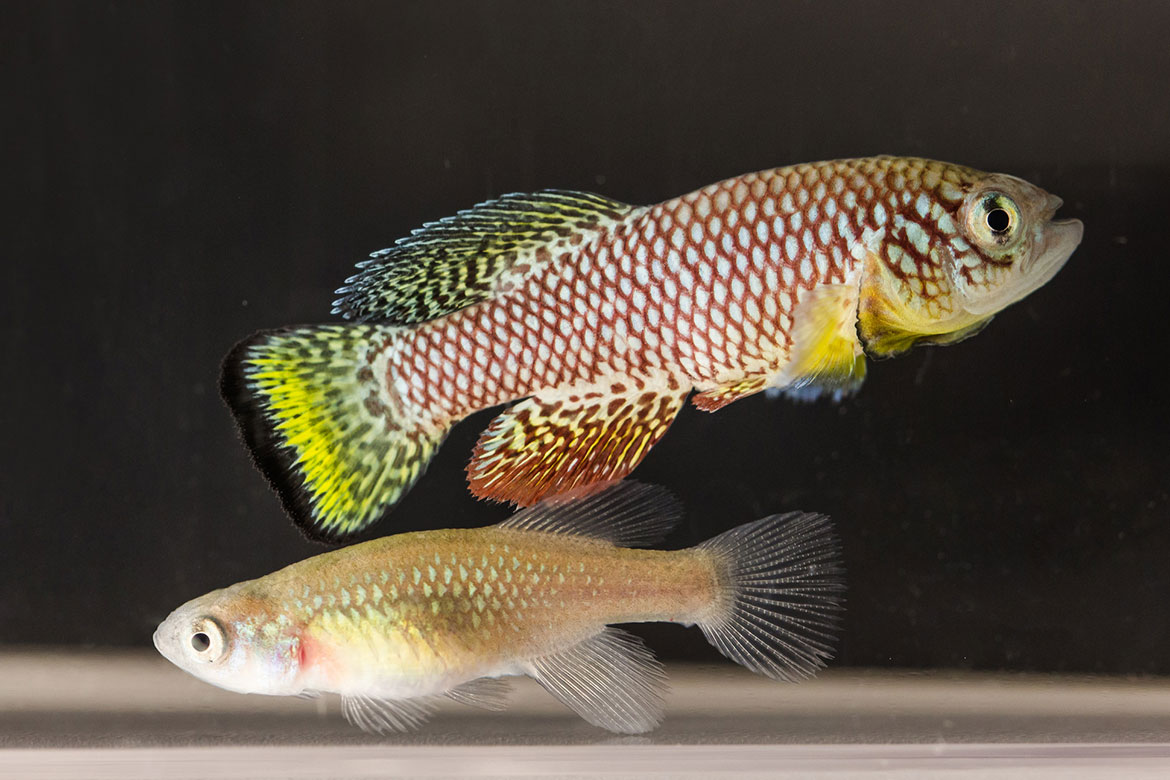
It reproduces quicker than mice: the killifish Nothobranchius furzeri. | Photo: Nadine Grimm
Geriatric at five months
In recent years, the killifish has become very popular with the research community. It ages extremely quickly – some laboratory strains have a maximum lifespan of 150 days. What’s more, its body shows clear signs of ageing. This little toothcarp from Africa is therefore ideal for investigating the ageing process. “We can conduct experiments in a much shorter space of time than is possible with standard vertebrate models such as the mouse”, says Alejandro Ocampo of the University of Lausanne, who brought the first-ever killifish colony to a Swiss laboratory just over a year ago.